The ultimate source of utility data
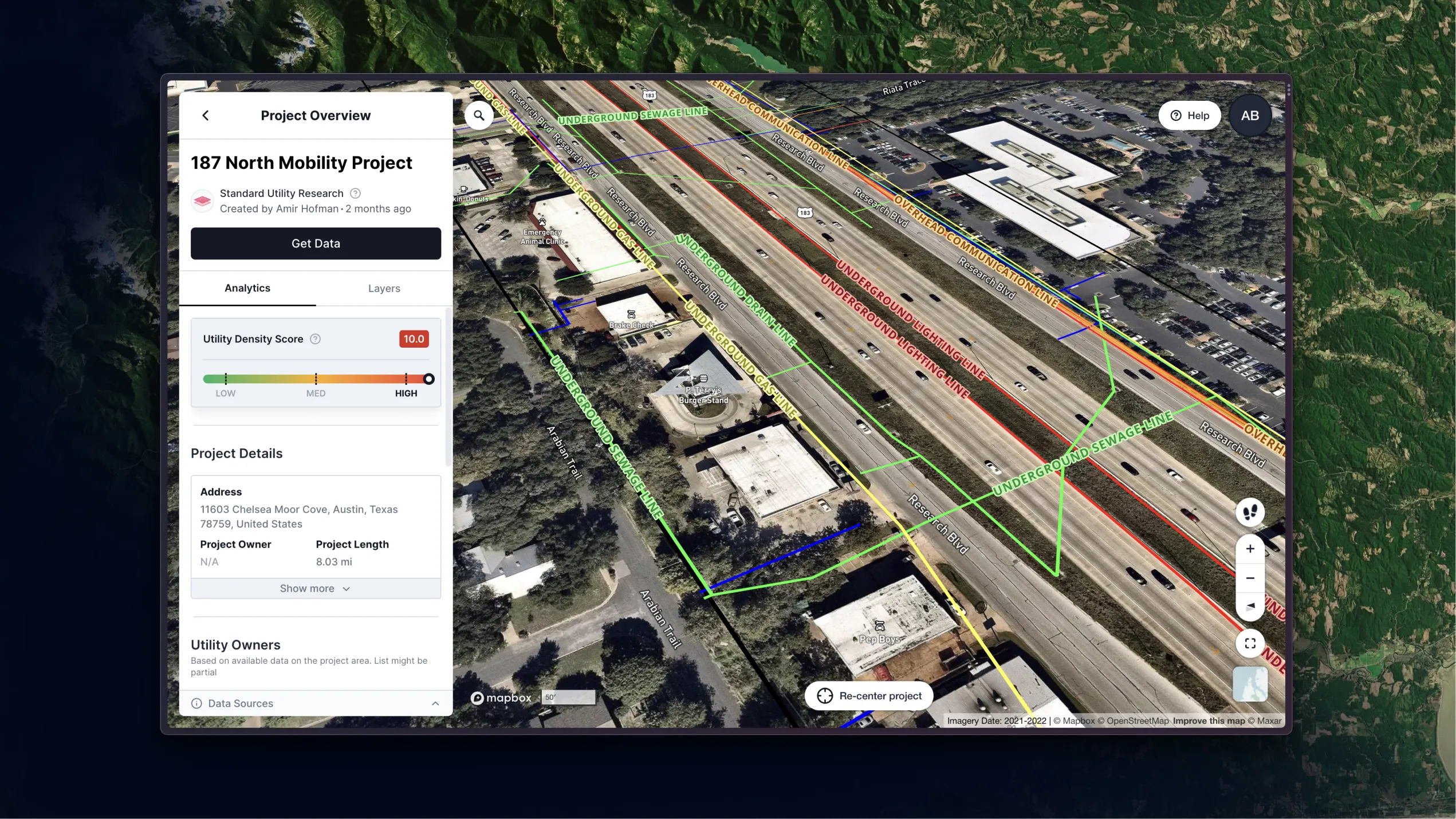
Start utility risk mitigation and coordination as early as pre-design. Without leaving the office. Without leaving your desk.
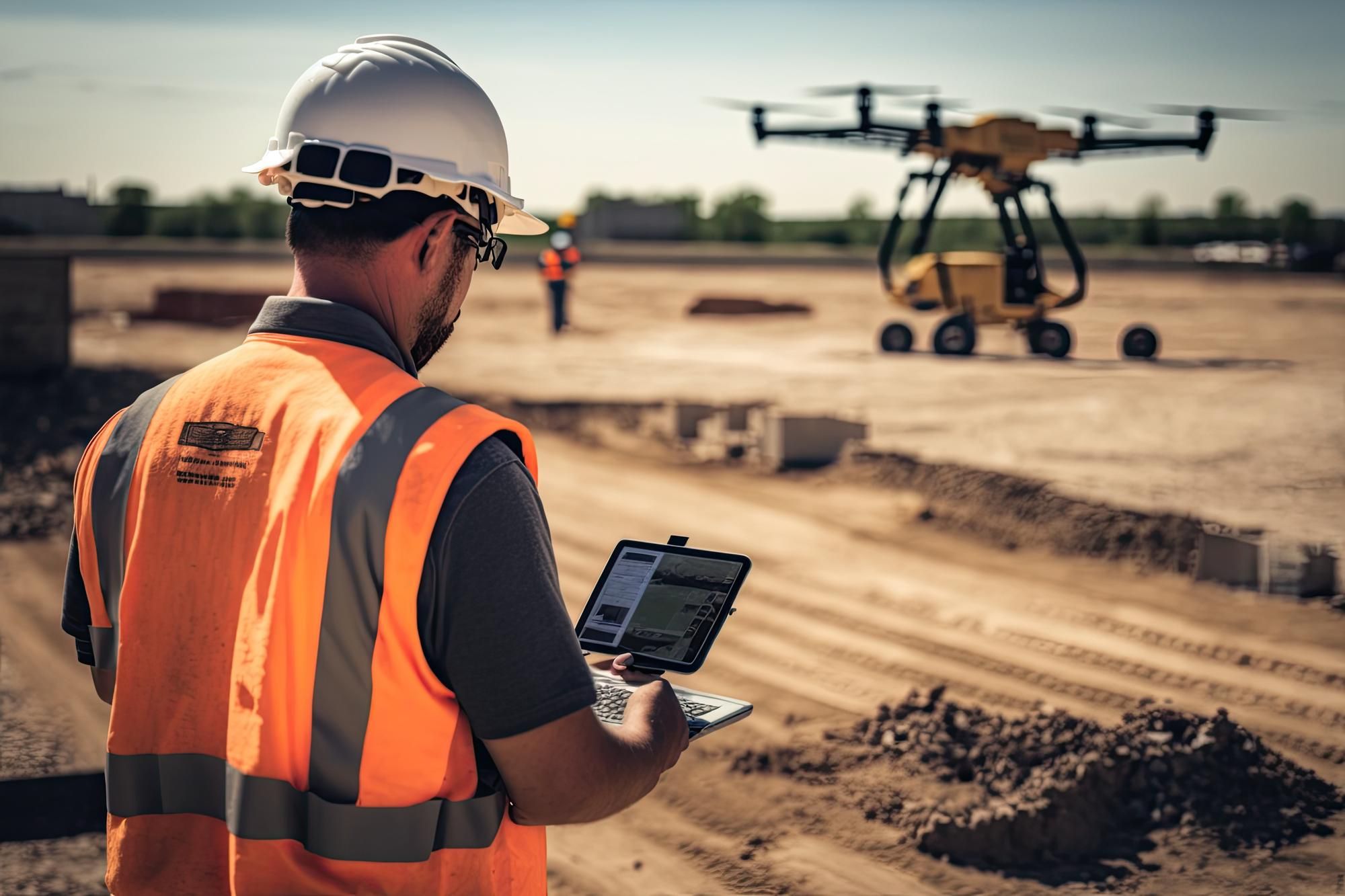
A Comprehensive Guide to Ground Penetrating Radar (GPR)
Introduction to Ground Penetrating Radar (GPR)
Ground Penetrating Radar (GPR) is a powerful subsurface exploration tool that uses electromagnetic waves to detect and map objects and structures beneath the ground's surface. GPR is useful for locating utilities because it detects what's beneath the surface without the expense and risk of digging or potholing.
"Relative to the liability of a utility strike, the cost of a GPR scan is negligible," says Dr. Sean McConnel, geophysical archeologist at Bigman Geophysical and a recent guest on 4M's Everything Analytics Podcast. "For an expensive project, it's worth it to go ahead and do a scan before you break ground."
In this guide, we'll explore GPR applications, technology, pros and cons, and more. Use the links below to jump to what you need:
How does GPR work?
Applications of GPR
Understanding GPR technology and equipment
Data interpretation in GPR
Advantages and limitations of GPR
GPR vs. other imaging techniques
Significance of GPR frequency
Visualizing GPR data
Future trends in GPR technology
How much does GPR cost?
How does GPR work?
GPR works by detecting echoes of electromagnetic signals. First, the transmitter sends high-frequency radio waves (between 10 MHz and 2.6 GHz) into the ground. The waves pass through objects under the surface and are distorted by conductive and dielectric properties. Then, the reflecting pulse returns to the GPR receiver, which reads the signal distortions, displaying contrasts between objects below the surface along with depth information.
GPR software can interpret that data to create a map of what is underground. For example, a PVC pipe will have a different electromagnetic profile than a copper wire, and the technology can distinguish between those without the need to dig.
Applications of GPR
GPR finds applications in a wide range of industries, making it a versatile tool for subsurface exploration. In construction, GPR is used for locating utilities, assessing concrete structures, and identifying potential hazards before excavation. For example, GPR was used to detect the location of a stormwater sewer beneath an existing metropolitan area, allowing for the safe installation of fiber lines with minimal digging.
Geologists can use GPR to determine soil properties and identify types of minerals found under the surface. This application is useful for surveying potential construction sites, finding fresh groundwater sources, and assessing the risk of future landslides. For example, GPR was used to analyze water and landslide risk after a mine closed in Brazil, and to determine the probability of future disasters after a 2010 landslide in Poland.
In archaeology, GPR can detect long-buried evidence of past civilizations, including buried structures, underground tunnels, military equipment, and human remains. For instance, archeologists used GPR to find the remains of the Württemberg-Stambol Gate in Belgrade, Serbia, preventing a construction crew from destroying an archeological site.
Environmental scientists use GPR to evaluate the impact of waste on the environment — for example, to see how far contamination from a landfill has spread or find buried waste material that needs to be removed. GPR was used successfully to detect lead contamination from battery disposal in Indonesia.
GPR also has applications in law enforcement for finding forensic evidence beneath the surface, from human bodies to buried illegal drugs or weapons. In Italy, the technology was used successfully to locate the body of a suspected murder victim hidden in an underground cave.
Military personnel use GPR to detect potentially deadly explosives such as undetonated mines, allowing them to dismantle them before they are disturbed and cause harm. Government entities have also discussed the value of using GPR to detect illegal objects at nuclear facilities and enhance subterranean physical protection systems.
Understanding GPR technology and equipment
While the appearance of different models may vary, the typical GPR unit looks like a push-mower with a digital screen attached to the handle. GPR systems consist of several essential components, including a transmitter, receiver, and control unit.
The transmitter sends electromagnetic signals into the subsurface, creating echoes that the receiver (normally the larger section that rolls along the ground) then detects.
The control unit, or interface through which the operator runs the device, then interprets the echoes with the help of software and mapping tools.
Antennas play a crucial role in GPR's subsurface penetration. Both the transmitter and receiver are types of antennas, and they utilize different frequencies depending on the desired depth of investigation and the type of material being scanned. Low-frequency antennas provide greater depth penetration but lower resolution, while high-frequency antennas offer higher resolution but shallower penetration. The size of the detectable object also varies depending on depth — while a small object can be detected in shallow earth, it would be undetectable at a deeper position.
Data interpretation in GPR
GPR data collection involves scanning the ground in a grid pattern, capturing reflections from subsurface features. The amplitude and wavelength of the electromagnetic pulses change as waves travel through materials with different physical or chemical properties.
The collected data is typically presented in the form of radargrams, which display the amplitude of reflected signals over time.
Interpreting GPR data requires expertise and an understanding of subsurface features. Various techniques, such as hyperbola fitting and waveform analysis, are used to identify subsurface objects, differentiate between materials, and map geological structures.
But data interpretation in GPR can present challenges. For example, if the electromagnetic properties of soil are unknown, they can distort signals and lead to poor data. Target resonance, or how the signals interact with objects, can lead to false readings that show more objects than actually exist. (For example, if the material of a single pipe interacts in an unexpected way, it might show up as three different pipes in the GPR reading.)
Because factors like signal attenuation, interference, and complex subsurface conditions can affect the accuracy of interpretations, it's crucial to consider these challenges and employ appropriate techniques to ensure reliable results.
Advantages and limitations of GPR
GPR offers several advantages that make it a valuable tool for subsurface exploration. It is non-invasive, meaning it circumvents the cost, environmental disruption, and time it takes to physically dig. It's also quick and easy for a trained technician to image a large area, yielding real-time results for immediate analysis and decision-making. While the size of the device dictates how far it can detect objects underground, most GPR products are small and easy to handle — ranging from handheld to lawnmower-size units.
However, GPR also has limitations. A big one is the issue of depth constraints, influenced by factors such as soil conditions, electrical conductivity, and antenna frequency. While GPR can see up to 100 feet underground in perfect conditions, the deeper you go, the lower the resolution will be — and the deeper you need to scan, the larger the device needs to be.
"When you find linear features, especially metal objects, most of them are very easy to see," says Dr. McConnel. "When you get deep enough, though, you might get phantom images."
GPR also requires proper soil composition that's conducive to the electromagnetic pulses it uses to gather data. Highly conductive materials, such as clay or water, can limit the penetration depth of GPR, and the amount of water in the ground can also limit how far down the device can detect objects.
GPR also cannot determine the composition of a target object — only the contrast between it and its surroundings. If an object has a similar composition to the soil around it, it might be missed.
While GPR products are typically easy to operate, correctly interpreting the data requires a lot of training because of all the variables involved.
GPR vs. other subsurface imaging techniques
How is GPR different from other subsurface imaging techniques?
Sonar
While some have attempted to use sonar to see underground structures, this technology needs water to work properly because it uses sound waves rather than radio or electromagnetic waves.
Seismic methods
Known for their use in earthquake detection and measurement, seismographs measure seismic waves moving through the earth's crust. The waves can be naturally occurring (such as in a tectonic plate shift) or generated, and are analyzed in a way similar to GPR to determine the presence of mines, types of bedrock, and other underground features. But seismic methods are far less accurate than GPR when it comes to identifying subsurface objects, and can only detect large structures.
Traditional excavation
Hand digging or potholing is, of course, the most reliable way of locating objects underground — but it's also the slowest and costliest.
When comparing GPR with other subsurface imaging techniques, such as sonar, seismic methods, and traditional excavation, GPR stands out for its unique strengths — high-resolution imaging, allowing for detailed subsurface mapping and the ability to locate metal objects as small as a few centimeters wide. GPR is also faster and more cost-effective than traditional excavation methods, reducing time and resources required for subsurface investigations.
"GPR has become the gold standard," says Dr. McConnel. "It's the equipment that has probably the lowest failure rate and the highest confidence in its results."
Significance of GPR frequency
The frequency of GPR antennas plays a crucial role in the interaction of electromagnetic waves with the ground.
Differences in antenna frequencies
Low-frequency antennas, typically ranging from 100 to 400 MHz, are suitable for deep subsurface investigations, such as locating utilities or studying geological formations. Medium-frequency antennas, around 400 to 1000 MHz, strike a balance between depth and resolution, making them ideal for general-purpose GPR surveys.
High-frequency antennas, ranging from 1000 to 3000 MHz, provide excellent resolution but limited depth penetration. They are commonly used for detailed concrete inspections, archaeological surveys, and detecting shallow subsurface objects.
Visualizing GPR data
GPR data can be visualized using various methods to enhance interpretation and understanding.
Radargrams, which display the amplitude of reflected signals over time, provide a detailed view of subsurface features.
Time-slice maps offer a plan view of subsurface structures at different depths, providing a comprehensive understanding of the site.
Three-dimensional imaging techniques, such as tomography and volumetric rendering, allow for a more immersive visualization of subsurface features.
Effective data presentation is crucial for clear insights and informed decision-making.
Future trends in GPR technology
The future of GPR technology holds exciting possibilities. Advancements in data processing algorithms will enhance the accuracy and efficiency of GPR data interpretation, even at greater depths. Recent research shows that integrating the traditional linear model of data analysis with newer, nonlinear filtering and image reconstruction will offer better imaging that's easier for operators to interpret.
Miniaturization of equipment is another innovation in the works. Traditionally, depth perception in GPR has been associated with the size of the scanning device — the larger the antenna, the deeper it can see. But recent research involving magnetic antennas reveals that antenna size can be greatly reduced, creating highly portable and accessible GPR systems that can penetrate 100 meters or more, depending on soil conditions.
Integrating GPR with other technologies is another emerging advancement — for example, combining GPR with augmented reality can allow workers and planners to see images of underground pipes, wires, or other structures overlaid on a surface image.
Used in conjunction with GPS, GPR data can be recorded with hyper-accurate location information — an imperative tool when precision matters on a construction site.
"In the future, we'll see more and more auto-detection, and more augmented reality, which is really powerful," Dr. McConnel says. "It means the guy in the excavator can see those underground objects from his phone. We're getting to a point where GPR data is eminently more sharable and faster to process."
Here's how these innovations might impact industries and applications:
Improved data
With better imaging that's easier to interpret, GPR operators would not need as much training — cutting down on labor shortages in the construction industry.
Miniaturization
Transporting a GPR unit to a remote location would be much simpler if the unit is very small — or attached to a drone or robotic vehicle. This versatility could impact many use cases, from construction to law enforcement to archeology.
Integration with augmented reality and GPS
Combined with augmented reality and GPS, GPR could allow military personnel to see buried mines and avoid them. Environmental scientists could use a combination of GPR and GPS to track the movement of underground contaminants over time.
How much does GPR cost?
The cost of GPR can vary depending on several factors such as the size of the area to be scanned, the depth of the subsurface features, and the complexity of the project.
Currently, the cost of GPR equipment ranges from a few thousand dollars for a basic handheld device to tens of thousands of dollars for a more advanced system — and there may be additional costs for accessories such as antennas, software, and data analysis tools.
GPR surveys are typically priced based on the area to be scanned and the depth of the subsurface features. Prices can range from a few hundred dollars for a small residential project to several thousand dollars for a larger commercial or industrial project.
Another option is gathering data and sending it to a professional for interpretation. Consulting fees for interpreting GPR data might be a few hundred dollars per hour, but will yield much better results than untrained locators attempting to interpret the data.
It's important to note that these costs are estimates and can vary depending on the specific requirements of the project and the location. For more accurate cost estimates, contact GPR service providers or equipment manufacturers.
Conclusion
GPR is a powerful tool for subsurface exploration, offering non-invasive, real-time imaging of subsurface structures and objects. Its applications span across industries, from construction to archaeology, geology, and environmental science.
As advancements in GPR technology continue to unfold, the future holds promising possibilities — and the industry is growing.
"There are going to be a lot more GPR techs in 2025 than there are right now," Dr. McConnel says.